Team:MIT/Projects/Project2
From 2009.igem.org
Light-inducible protein localization in Yeast
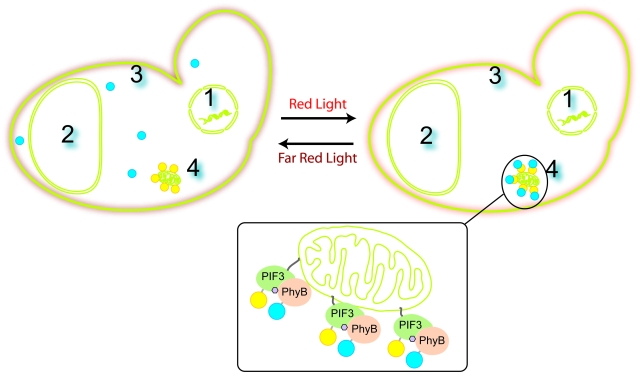
Objective
We set out to create a quick, reversible switch to control the localization to various targets within a yeast cell, such as the nuclear membrane, plasma membrane, mitochondrial membrane, and vacuole.
Motivation
To fully control cellular functioning, it would be useful to have a modular system that can provide both temporal and spatial control over any protein of interest within a cell. In other words, we should be able to control both when a gene is expressed and where the protein is functioning. The existing PhyB-PIF3 system provides temporal control over gene expression by acting as a transcriptional regulator. However, by adapting the PhyB-PIF3 system to control protein targeting, we can have both temporal and spatial control over a protein's functioning. Such a system can potentially be used as an on-off switch for essential genes by controlling movement the protein to and from where the protein functions in the cell. In addition, because protein targeting is key in controlling the cell cycle, the system can also potentially be used to control cell cycling and differentiation. Furthermore, reversible control of protein localization and delocalization can be useful as a tool to study diffusion rates within a cell.
Background on PhyB-PIF3 System
The light switching mechanism that we use exploits the machinery by which plants and algae respond to varying light conditions. Phytochromes are light sensitive proteins that are composed of a polypeptide bound to a small pigmented molecule called a chromophore. They have two conformations: the inactive Pr form and the active Pfr form. When red light is present, the phytochrome switches from the Pr to the Pfr form. When in the Pfr form, the phytochrome can bind to proteins called phytochrome interacting factors. In nature, the resulting complex can bind to DNA and act as a transcriptional regulator. When far red light is present, the phytochrome dissociates from the phytochrome interacting factor, making the switching mechanism reversible. Peter H. Quail's lab at University of California Berkeley developed a synthetic biological switch using a phytochrome called PhyB, which is bound to a chromophore called phycocyanobilin (PCB). Red light causes PhyB to bind to a phytochrome interacting factor called PIF3, and far-red light causes them to unbind. Our system harnesses this switching mechanism to control post-translational processing instead of transcriptional regulation.
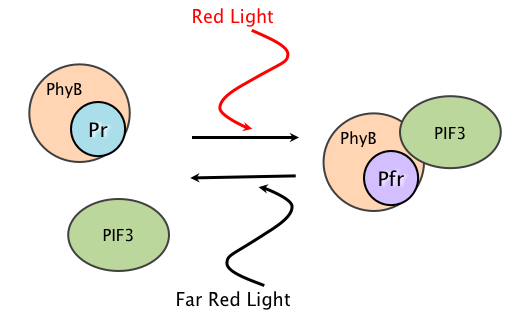
Mechanism
To localize our protein of interest, either PhyB or PIF3 is anchored to the target while the other is the traveler construct that diffuses throughout the cell. The anchored construct contains a signal sequence, which causes it to be localized constitutively to the target. The traveler, on the other hand, is fused to the protein of interest. When pulsed with red light, the PhyB will switch to the Pfr conformation. As a result, the as the traveler diffuses throughout the cell, PhyB and PIF3 will bind, which attaches the traveler to the anchor. As the traveler becomes bound to the anchor, the protein of interest becomes localized to the target. Under far red light, the anchor and the traveler dissociate, the the protein of interest again can diffuse throughout the cell. In our system, PIF3 is tagged with YFP and PhyB is tagged with CFP so that the localization and delocalization can be observed using fluorescence microscropy.
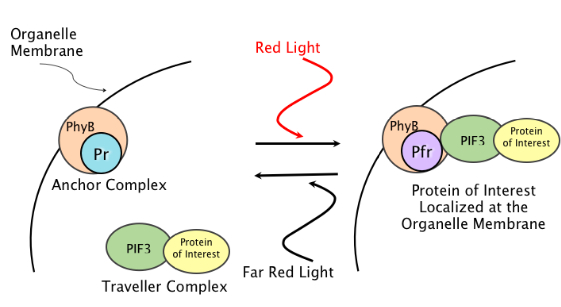
Constructs
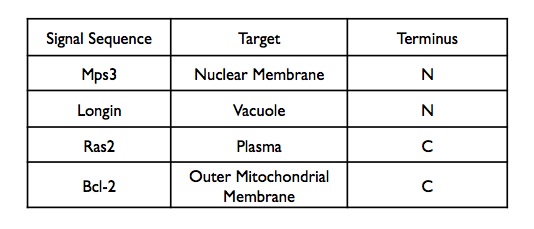
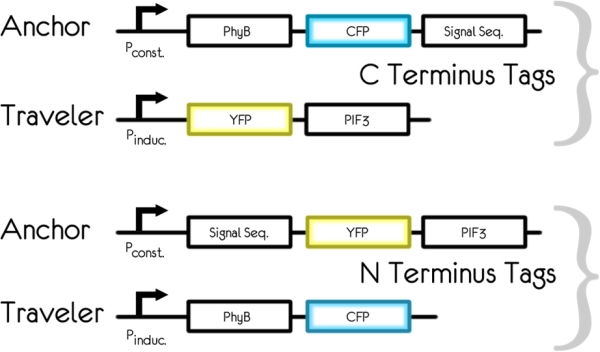
Materials and Methods
Construct Construction
Sequential Transformation Plasmid Construction
Construction of the PhyB-CFP and Pif3-YFP Plasmids began with the amplification of each of the individual sequences, PhyB, CFP, PIF3 and YFP, from the Berkley Lab Plasmids. These sequences were subsequently digested and ligated into the prS313 backbone, a plasmid that contains a constitutive Myo2 promoter and the His gene. PIF3 and CFP were inserted using NotI and SacII, while PhyB, YFP used NotI and XmaI. Once the plasmid was complete it was transformed into E. coli. The PhyB and Pif3 plasmids transformed successfully, which was confirmed through a check digest and sequencing. The corresponding fluorescent protein DNA was then incorporated into the plasmid through another round of digestion, ligation and transformation. The sequencing results for these plasmids were positive for incorporation of both DNA pieces for both of the constructs. Therefore, each of these plasmids were transformed into yeast and observed for fluorescence through microscopy.
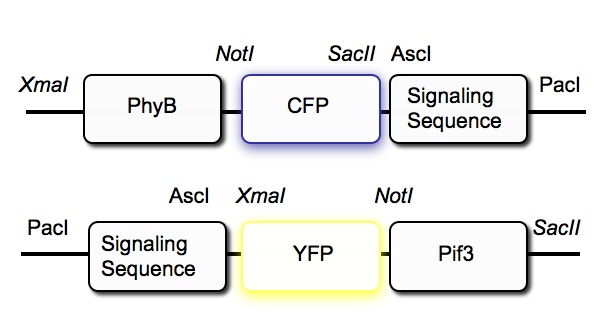
Caption: The construct design with Restriction Enzymes for Sequential Transformation.
Alongside work in the base constructs, a variety of C and N terminus localization sequences were chosen to test the PhyB, Pif System. Each localization sequence, once amplified, would be cloned into the appropriate backbone using the restriction enzymes. N terminus tags were destined for the Pif3-YPF backbone and C terminus for the PhyB-CFP Backbone
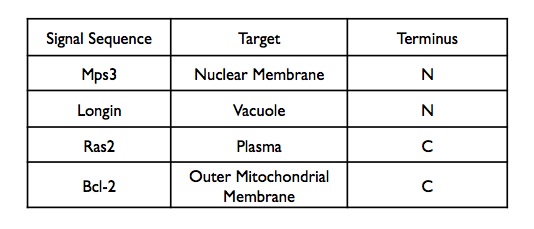
Caption: A table of the signaling sequences chosen. These would serve to anchor one protein to a particular organelle.
Homologous Recombination
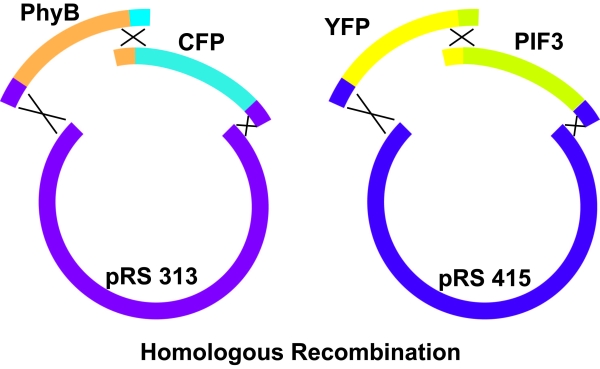
Caption: A depiction of the plasmids into which the PhyB-CFP and PIF3-YFP were inserted.
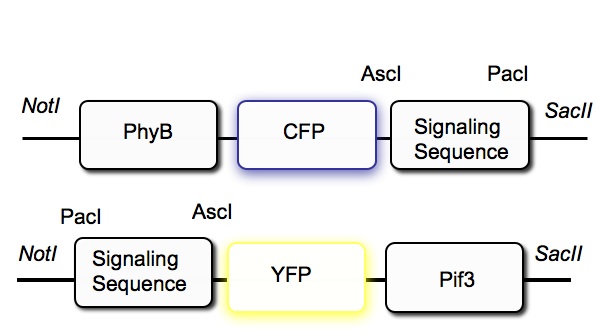
Caption: The construct design with Restriction Enzymes for Homologous Recombination.
LED Arrays for Red and Far Red Light
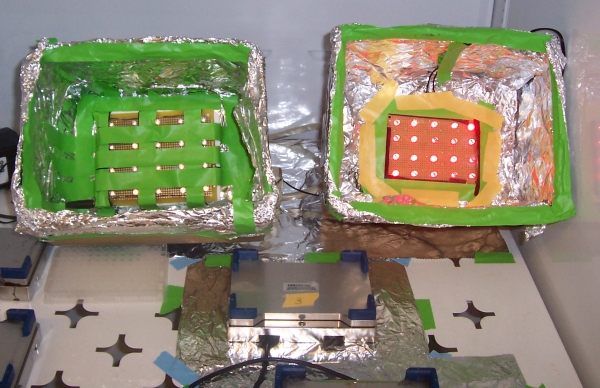