Team:HKU-HKBU/Speed Control Design
From 2009.igem.org
(→Design) |
(→Design) |
||
Line 5: | Line 5: | ||
=Design= | =Design= | ||
- | Speed control is a crucial feature of our Bactomotor and is indispensable for more advanced controllable applications. Devices equipped with the speed controllable Bactomotor open up possibilities of non-invasive micro-surgery. Obviously, we may not expect the bacteria motor to behave exactly according to our will. After all, our motor is alive! It is subjected to numerous physical and physiological limitations | + | Speed control is a crucial feature of our Bactomotor and is indispensable for more advanced controllable applications. Devices equipped with the speed controllable Bactomotor open up possibilities of non-invasive micro-surgery. Obviously, we may not expect the bacteria motor to behave exactly according to our will. After all, our motor is alive! It is subjected to numerous physical and physiological limitations. But the capacity of tweaking the swimming speed greatly promotes its usefulness. In the case of micro-surgery, we can slow down the Bactomotor in order to locate the pathologic tissue. We can then increase the bacteria to its full speed to bring desirable mechanical changes to the target area. Another example that will illustrate the importance of speed control is in the case of drug delivery. On one hand, we may wish to make the drug-loaded Bactomotor to swim faster than it normally does to overcome the resistance it encounters with in the capilliaries during the process of delivery; on the other hand, we wish to slow down the Bactomotor in time to allow more accurate localization of drug. |
''E. coli'' or ''Salmonella'' can swim around by rotating the flagella. When the flagella rotate in a counterclockwise fashion, the bactomotor gathers momemta and produce non-random locomotion. When the rotation is in the clockwise direction, the bactomotor will tumble in place and fail to 'swim' (Fig 1). | ''E. coli'' or ''Salmonella'' can swim around by rotating the flagella. When the flagella rotate in a counterclockwise fashion, the bactomotor gathers momemta and produce non-random locomotion. When the rotation is in the clockwise direction, the bactomotor will tumble in place and fail to 'swim' (Fig 1). | ||
Line 13: | Line 13: | ||
- | To control the speed of our Bactomotor, we aim at the direct swimming of bacteria for propelling the motor and the adjustable speed of swimming within a certain range. The aim is achieved by regulation of the expression level of cheZ gene. cheZ plays the key role here | + | To control the speed of our Bactomotor, we aim at the direct swimming of bacteria for propelling the motor and the adjustable speed of swimming within a certain range. The aim is achieved by regulation of the expression level of cheZ gene. The gene of cheZ plays the key role here as it influences the expression level of cheY. A high level phosphorylation of cheY protein in ''E. coli'' or ''Salmonella'' leads to the majority of bacteria tumbling movement, while a low level of phosphorylation of cheY protein in ''E. coli'' or ''Salmonella'' is found in non-tumbling bacteria and cheZ can dephophorylate cheY. Therefore, when increasing the expression level of CheZ gene, we can reduce the tumbling movement, which in turn can increase the swimming speed of the bacteria to achieve the manipulation of speed. |
+ | Speed control is not achieved by a single bacterium; On the contrary, it is the result of the change of behavior | ||
=='''Step 1--''CheZ'' knockout'''== | =='''Step 1--''CheZ'' knockout'''== | ||
Revision as of 16:10, 20 October 2009
Contents |
Design
Speed control is a crucial feature of our Bactomotor and is indispensable for more advanced controllable applications. Devices equipped with the speed controllable Bactomotor open up possibilities of non-invasive micro-surgery. Obviously, we may not expect the bacteria motor to behave exactly according to our will. After all, our motor is alive! It is subjected to numerous physical and physiological limitations. But the capacity of tweaking the swimming speed greatly promotes its usefulness. In the case of micro-surgery, we can slow down the Bactomotor in order to locate the pathologic tissue. We can then increase the bacteria to its full speed to bring desirable mechanical changes to the target area. Another example that will illustrate the importance of speed control is in the case of drug delivery. On one hand, we may wish to make the drug-loaded Bactomotor to swim faster than it normally does to overcome the resistance it encounters with in the capilliaries during the process of delivery; on the other hand, we wish to slow down the Bactomotor in time to allow more accurate localization of drug.
E. coli or Salmonella can swim around by rotating the flagella. When the flagella rotate in a counterclockwise fashion, the bactomotor gathers momemta and produce non-random locomotion. When the rotation is in the clockwise direction, the bactomotor will tumble in place and fail to 'swim' (Fig 1).
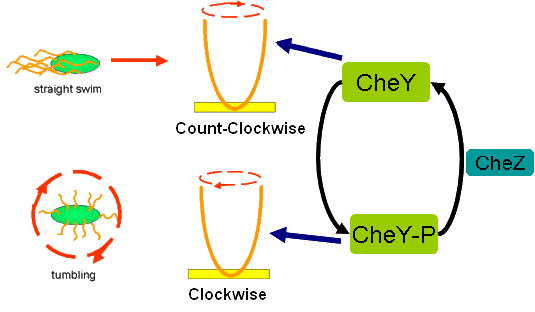
To control the speed of our Bactomotor, we aim at the direct swimming of bacteria for propelling the motor and the adjustable speed of swimming within a certain range. The aim is achieved by regulation of the expression level of cheZ gene. The gene of cheZ plays the key role here as it influences the expression level of cheY. A high level phosphorylation of cheY protein in E. coli or Salmonella leads to the majority of bacteria tumbling movement, while a low level of phosphorylation of cheY protein in E. coli or Salmonella is found in non-tumbling bacteria and cheZ can dephophorylate cheY. Therefore, when increasing the expression level of CheZ gene, we can reduce the tumbling movement, which in turn can increase the swimming speed of the bacteria to achieve the manipulation of speed.
Speed control is not achieved by a single bacterium; On the contrary, it is the result of the change of behavior
Step 1--CheZ knockout
By using lamda red system, recombineering is utilized to knock out the CheZ gene in the chromosome of E. coli or Salmonella. Homologous arms (about 50bp)are placed inside the CheZ gene. The CheZ gene is replaced by a chloramphenicol resistance gene after recombination.
Step 2--Controllable cheZ expression
An inducible cheZ plasmid was tranformed into CheZ knockout strains. Therefore, by controlling cheZ expression level, we can implement the adjustable control over the speed of the bacteria and hence the motor.
There are two designs for cheZ plasmid.
Original Design
The orinigal design is to use lacI as a repressor to prevent the occurence of leaky expression in the absence of the inducer, which in this case is IPTG(Isopropyl β-D-1-thiogalactopyranoside). We predict that the bacterium will swim at a lower speed when it is in a 'incomplete tumbling mode'. When the bacteria are treated with IPTG(switch on), the expression level of cheZ could be regulated according to inducer's concentration and hence swimming speed of the bacteria.
Back up Design
The back up design is to use tetR as a repressor and ptet as the regulator, which can be induced by tetracycline(or aTc). We suppose that by changing the concentration of tetracycline, the expression amount of protein cheZ will be altered, resulting in the acceleration and deceleration.